Horizontal Range Horizontal range: It is the horizontal distance travelled by a body during the time of flight. So by using second equation of motion
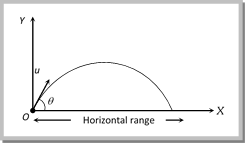
\[R=u\cos \theta \times T\]\[=\,\]\[u\cos \theta \times (2u\sin \theta /g)\]\[=\frac{{{u}^{2}}\,\sin \,2\theta }{g}\] \[R=\frac{{{u}^{2}}\,\sin \,2\theta }{g}\] (i) Range of projectile can also be expressed as: \[\operatorname{R} = u cos\,\theta \,\,\times \, T =u\cos \theta \frac{2u\sin \theta }{g}=\frac{2\,u\cos \theta \,\,u\sin \theta }{g}=\frac{\text{2}{{\text{u}}_{\text{x}}}{{u}_{y}}}{\text{g}}\] \ \[R=\frac{\text{2}{{\text{u}}_{\text{x}}}{{u}_{y}}}{\text{g}}\] (where \[{{\operatorname{u}}_{x}}\,and {{u}_{y}}\] are the horizontal and vertical component of initial velocity) (ii) If angle of projection is changed from \[\theta \] to \[\theta =(90\theta )\] then range remains unchanged.
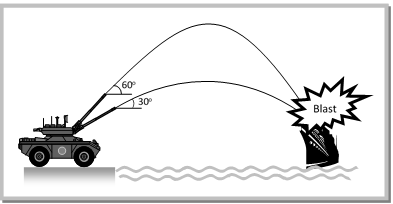
\[R'=\frac{{{u}^{2}}\sin 2\theta \,'\,}{g}=\frac{{{u}^{2}}\sin [2({{90}^{o}}-\theta )]}{g}=\frac{{{u}^{2}}\sin 2\theta \,}{g}=R\] So a projectile has same range at angles of projection q and (90 ? q), though time of flight, maximum height and trajectories are different. These angles q and \[{{90}^{o}}\theta \]
more...